Quantum Builders Summit Taiwan 2025
2025.01.13
Author:Peir-Ru Wang
\(\to\)中文版
- Event Introduction
- Reliable qubits
- Real-time calibration and control signals
- Collaboration with classical computers
- Simulation and fitting of control parameters
-
Effective error correction
- Hardware: Error correction circuits
- Software: Error correction algorithms
- Towards Robust Entanglement in Scaling up Connectivity of Superconducting Qubits
- 1 Qubit characterization: Measure operational parameters of each qubit and quantum circuit
- 1 Qubit parameter determination and calibration
- 2 Qubit parameter determination and calibration
- Device and Technology Co-Design of Germanium Quantum Dots/Si-based Barriers Integrable Charge Qubits
- Building Scalable Quantum Computers with Silicon MOS Qubits
- Accelerated Quantum Supercomputing
- OPX1000 Demo
Quantum computing demonstrates immense computational potential in specific problems compared to classical computers. However, its hardware faces challenges such as control difficulty and short operation times. A complete quantum computing system must rely on:
Professor Chii-Dong Chen from Academia Sinica clearly demonstrated the multiple aspects required to integrate and achieve a quantum computer:
▲Ref: Prof. Chii-Dong ChenRegarding the realization of qubits, there are still many different methods under development, each with its own strengths and weaknesses. Professor Pei-Wen Li from NYCU presented the characteristics of four mainstream types of qubits:
▲Ref: Prof. Pei-Wen LiQuantum computing is still in its developmental stage and requires the integration of multiple teams from different fields to achieve success. Therefore, Quantum Machines organized the Quantum Builders Summit Taiwan 2025, inviting professionals from academia and industry for collaboration and education. The presentation topics are as follows:
Subject | Speaker | From |
---|---|---|
Qubits | ||
Towards Robust Entanglement in Scaling up Connectivity of Superconducting Qubits | Prof. Chii-Dong Chen | Academia Sinica |
Device and Technology Co-Design of Germanium Quantum Dots/Si-based Barriers Integrable Charge Qubits | Prof. Pei-Wen Li | NYCU |
Building Scalable Quantum Computers with Silicon MOS Qubits | Dr. Henry Yang | Diraq |
Quantum Computing | ||
Accelerated Quantum Supercomputing | Mr. Yun-Yuan Wang | NVIDIA |
Quantum Error Correction | ||
The Case of the Misbehaving Superconducting Qubits | Dr. Shai Machnes | Qruise |
Building a Hardware-Software Pipeline for Fault-Tolerant Quantum Error Correction | Dr. Tommaso Demarie | Entropica Labs |
Qubit Control | ||
Hybrid Quantum Control | Dr. Itamar Sivan | Quantum Machines |
OPX1000 Demo | Dr. Jonathan Reiner | Quantum Machines |
Prof. Chii-Dong Chen
Academia Sinica
Prof. Chen’s talk focused on the efforts of Academia Sinica in Taiwan to develop and apply superconducting quantum computers. The construction of a quantum computing and production line center in Tainan is nearing completion. To achieve superconducting qubit-based computations, high-quality superconducting circuits need to be fabricated, followed by a series of calibration processes:
▲ Each major task requires a series of parameter measurements. Parameters across different qubits must be separated to avoid cross-talk. For example, qubits under the same drive line need to be set to different operational frequencies. If these frequencies are too close, it could lead to erroneous control effects.
▲ Example of 2 Qubit calibration. Multi-qubit circuits often required over two weeks for a complete calibration in the past. Furthermore, superconducting qubit parameters change over time, necessitating daily recalibration. While superconducting qubits are the most commonly adopted quantum computing hardware, their sensitivity to interference, errors, and decoherence is a significant challenge.
Prof. Pei-Wen Li
National Yang Ming Chiao Tung University (NYCU)
Prof. Pei-Wen Li specializes in quantum dots (QDs). Among mainstream qubit technologies, spin qubits—based on silicon or germanium materials to create localized quantum states with spin properties—are an important area. The spin direction is utilized for qubit operations.
▲ Due to the advanced manufacturing technologies and industrial expertise in silicon and germanium semiconductors, spin qubits based on these materials can leverage existing fabrication techniques, offering significant development potential.
▲ Localized quantum states for spin qubits can be implemented through gate-defined quantum dots (left) or "physical" quantum dots (right). Gate-defined quantum dots are created by applying voltages to electrodes deposited on silicon or germanium, forming potential wells. However, these require very stable voltage signals as even minor disturbances (considering the quantum scale of \(\hbar \approx 6.58 \times 10^{-16} eV\cdot s\)) can cause decoherence. On the other hand, physical quantum dots are formed through heterostructures but face challenges in integration and quality control.
▲ Quantum dots are essentially potential wells, where energy levels depend on the size of the dot. Using semiconductor technology, different devices can be fabricated. Qubits can be implemented with double quantum dots (DQDs) or as single-electron transistors (SETs). Flexible device designs enable the integration of quantum computational circuits with classical circuits.
Some references:
1. DOI: 10.1126/science.1118921 (Double Quantum Dots as a Quantum Bit)
2. Self-organized Pairs of Ge Double Quantum Dots with Tunable Sizes and Spacings Enable Room-Temperature Operation of Qubit and Single-Electron Devices
Dr. Henry Yang
Diraq
The previous section highlighted the advantage of silicon-based qubits in their compatibility with conventional semiconductor processes. Additionally, compared to superconducting qubits that require operating temperatures below \(0.1K\), silicon-based qubits have the potential to operate at around \(1K\), known as Hot Qubits. This significantly reduces dependence on low-temperature systems (though currently, photon-based quantum systems hold the most promise for room-temperature operation). Dr. Henry Yang’s presentation focused on achieving high-fidelity quantum control at \(1K\) and utilizing a universal algorithm to initialize 2-qubit states under any conditions (as shown below).
Mr. Yun-Yuan Wang
NVIDIA
Interestingly, just days before the event, NVIDIA CEO Jensen Huang remarked during the 2025 CES conference, “Quantum computing is likely 15–20 years away from maturity.”
Someday we'll have very useful quantum computers. We're probably five or six orders of magnitude away, 15 years for useful quantum computers and that would be on the early side. 30 years is probably on the late side. If you picked 20 years, a whole bunch of us would believe it. We want to help the industry get there as fast as possible and create the computer of the future.
While the statement suggests NVIDIA’s intent to accelerate quantum computing development, the timeline estimate quickly caused global quantum stocks to drop by 40%, leaving CEOs of companies like D-Wave frustrated.
The quantum realm is highly susceptible to noise, making it challenging to sustain accurate quantum computations for extended periods. Constant quantum error correction and hardware parameter adjustments are significant hurdles in quantum computing. For instance, Google’s study (*) on a superconducting quantum circuit comprising 72 qubits, 72 resonators, and 121 couplers required approximately 8,000 calibration parameters. A fault-tolerant quantum computer (FTQC) with evident computational advantages is estimated to require millions of qubits, with calibration parameters increasing exponentially. Leveraging AI for qubit control/readout, noise simulation, and reliability enhancement is crucial to advancing quantum computing.
(*) Ref: YouTube Video
Dr. Jonathan Reiner
Quantum Machines
Different qubits require distinct control protocols. Precise driving signals are essential for manipulating qubit states. Quantum control uses pulses of specific frequencies and waveforms, with amplitude adjustments to control quantum state transitions. The ideal waveform is shown below:
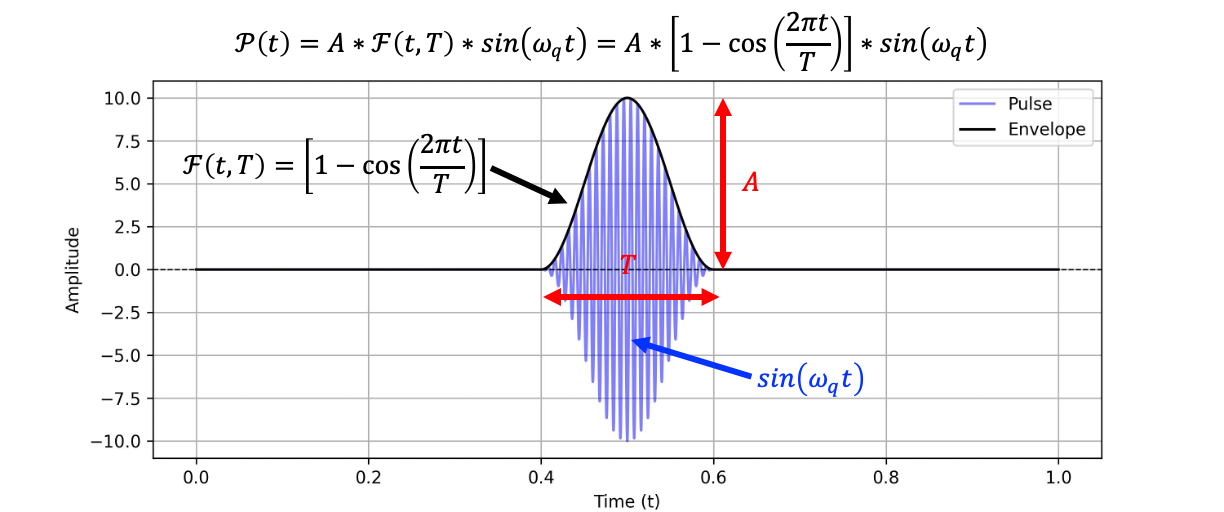
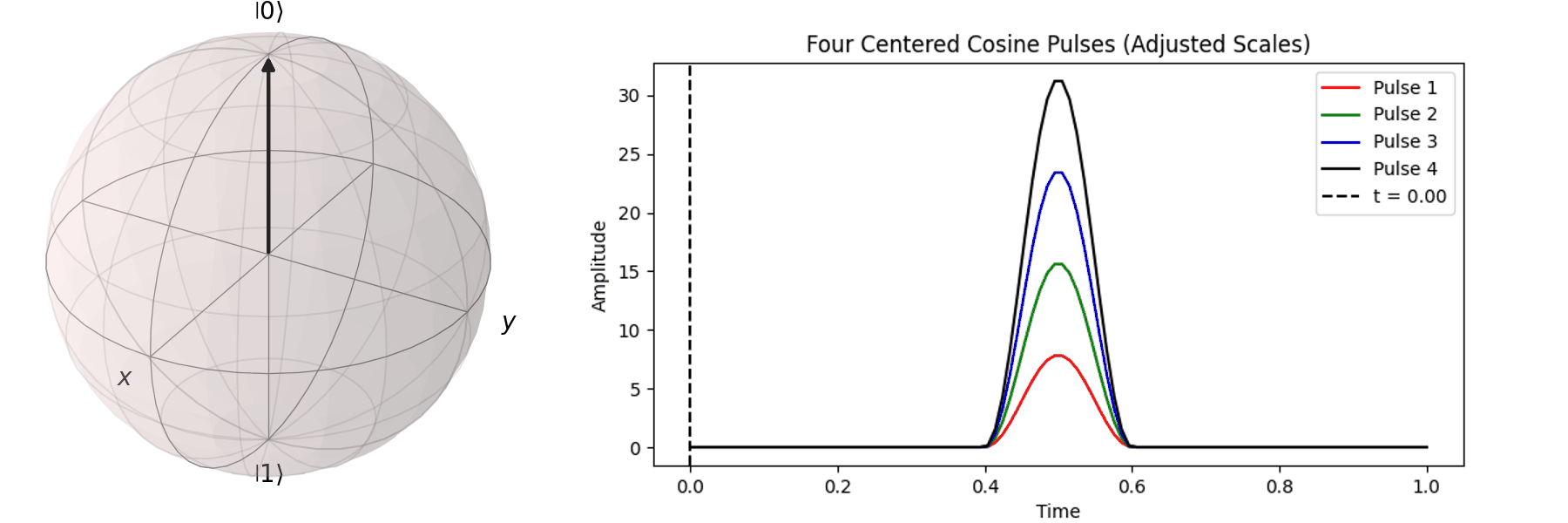
▲ An animation illustrating changes in quantum states corresponding to amplitude adjustments.
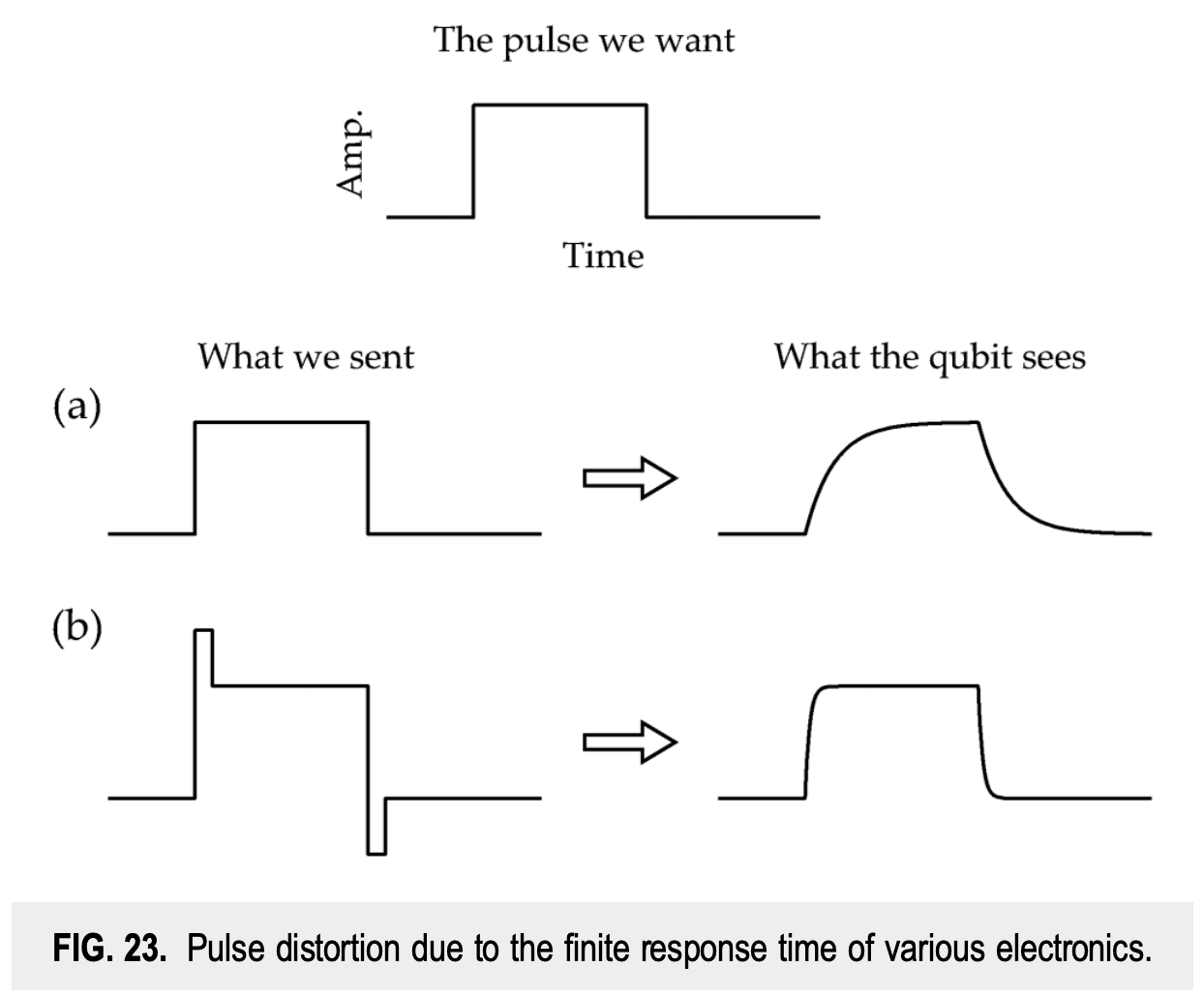
▲ In reality, these ideal pulse waveforms consist of a continuous frequency spectrum. Hardware limitations result in approximations using discrete frequencies, and interaction with the qubit often introduces signal distortions. Reference: DOI: 10.1063/5.0029735
▲ Quantum Machines demonstrated Power Rabi experiments using the OPX1000 control system. High-quality signal generation and robust electronic control equipment are indispensable components for realizing quantum computing.
Index
Contact: